Biochemical & Molecular Drought Tolerance Mechanisms in Plants (lec 8 & 9)
Phytohormones & growth regulators
- Under drought stress endogenous contents of Auxins, gibberellins & cytokinin usually decreases while those of ABA & ethylene increases
- Auxins induce new root formation by breaking root apical dominance induced by cytokinins.
- Auxins have indirect but key role in drought tolerance by inducing prolific root system.
- DS limits the production of endogenous auxins, usually when abscisic acid and ethylene increase.
- IAA (indole-3-acetic acid) enhance drought tolerance by increasing net photosynthesis and stomatal conductance.
- IBA (indole-3-butyric acid) a naturally occurring auxin
DS and ABA application——enhance IBA in maize
IBA synthesis is revealed as drought inducible in Arabidopsis
Drought Rhizogenesis (Role of gibbrellic acid (GA) in DR)
- Abscisic acid is a growth inhibitor and produced under a wide variety of environmental stresses, including drought.
- When plants wilt, abscisic acid levels typically rise as a result of increased synthesis, increased abscisic acid concentration leads to many changes in development, physiology and growth.
- All plants respond to drought and many other stresses by accumulating abscisic acid that induces the synthesis of Aminocyclopropane (ACP) carboxylate, which upon oxidation in the presence of O2 is converted to Ethylene (ETH). ETH inhibits the translocation of IAA and Auxin.
- Abscisic acid is ubiquitous in all flowering plants that regulates gene expression and acts as a signal for the initiation of processes involved in adaptation to drought and other environmental stresses .
- It has been proposed that abscisic acid and cytokinin. have opposite roles in drought stress Increase in abscisic acid and decline in cytokinins levels favor stomatal closure and limit water loss through transpiration under water stress .
- Abscisic acid increases the root-to-shoot dry weight ratio, inhibition of leaf area development, early ripening of fruits and production of prolific and deeper roots.
The Zn deficiency may be helpful for plants to resist drought stress
Zn is required for the synthesis of tryptophan-a precursor of IAA synthesis.
The IAA deficiency induced senescence may be preventing the loss of water through transpiration.
- Polyamines: (plant growth & development under stress)
- Spermidine: overexpression of spermidine synthase in apple substantially improve the drought tolerance
- Spermidine and spermine in free form and putrescine in insoluble-conjugated form-—–in response to drought stress
2.5 Compatible solutes and osmotic adjustment
Compatible solutes are low-molecular-weight, highly soluble compounds that are usually nontoxic even at high cytosolic concentrations.
Generally they protect plants from stress through different means such as contribution towards
osmotic adjustment,
detoxification of reactive oxygen species
stabilization of membranes, and native structures of enzymes and proteins
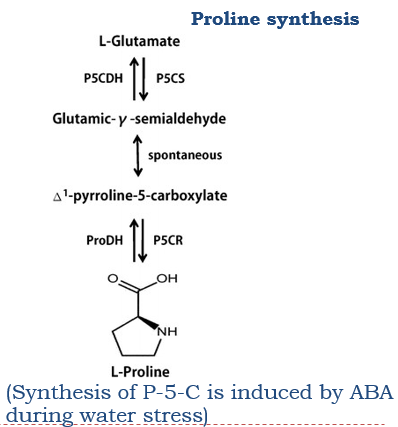
Compatible solutes are responsible for osmotic adjustment
Sugars and prolines protect lipid bilayer membranes from damage during cytoplasmic dehydration (osmoprotectant)
Intracellular/Ionic compartmentalization
Glycine Betaine (GlyBet)
3. Molecular Mechanisms:
- Plant cellular water deficit may occur under severe reduced soil water content, under such conditions changes in gene expression (up- and down-regulation) may take place.
- Various genes are known to induce under water stress at the transcriptional level and these gene products are thought to function in tolerance to drought.
- Gene expression can be triggered directly by the stress conditions or result from 2ndry stress or injury responses.
3.1 Aquaporins
- Aquaporins have the ability to facilitate and regulate passive exchange of water across membranes , when water flow needs to be adjusted or the flow is critically low.
- In plants, AQPs are present in almost all organs including roots, leaves, stems, flowers, fruits, and seeds
- They belong to a highly conserved family of major intrinsic membrane proteins.
- In plants, Aquaporins are present abundantly in the plasma membrane and in the vacuolar membrane.
- The structural analysis of aquaporins has revealed the general mechanism of protein-mediated membrane water transport.
- They can regulate hydraulic conductivity of membranes and potentiate a 10-20 fold increase in water permeability.
- Mercury is potential inhibitor of aquaporins, inducing decline in root hydraulic conductivity, which validated that AQP play a major role in overall root water uptake.
- Overexpression-PM Aquaporin in transgenic Tobacco-improved plant vigor
The plant aquaporin family
- Different plant AQPs have different substrate specificities, localization.
- Depending on membrane localization and amino acid sequence, AQPs in higher plants are classified into five subfamilies including
- Plasma membrane intrinsic proteins (PIPs)
- Tonoplast intrinsic proteins (TIPs)
- Nodulin-26 like intrinsic proteins (NIPs)
- Small basic intrinsic proteins (SIPs)
- X intrinsic proteins/uncharacterized-intrinsic proteins (XIPs)
- Plant species typically have a higher number of AQP genes than animals, ranging from 30 to 70.
- For example, in poplar, 55 AQP genes have been identified
Possible mechanisms for silicon (Si) mediated water balance of plants experiencing water deficiency.
(1) Si enhances the aquaporin activity by up-regulating the expression of Plasma membrane Intrinsic Protein (PIP) aquaporin genes and alleviating the ROS (reactive oxygen species)-induced aquaporin activity inhibition.
(2) Si enhances the accumulation of soluble sugars and/or amino acids in the xylem sap by osmorugulation;
Si activates the K + translocation to xylem sap by the activation the expression of SKOR (Stelar K + Outward Rectifer) gene. The osmolyte accumulations in the xylem sap increase the osmotic driving force.
(3) Si might adjust the root growth and increase root/shoot ratio, which together with enhancement of aquaporin activity and osmotic driving force contribute to the improvement of root hydraulic conductance. The higher root hydraulic conductance results in increased uptake and transport of water, which helps to maintain a higher photosynthetic rate and improve plant resistance to water deficiency.
3.2 Stress proteins
- Most of the stress proteins—-water soluble—-contribute in stress tolerance by hydration of cellular structures
- Membrane-stabilizing proteins, late embryogenic abundant proteins (LEA) and molecular chaperons (stabilizing other ‘proteins’ structure) are responsible for conferring drought tolerance.
- These increase the water binding capacity by creating a protective environment for other proteins or structures, referred to as dehydrins.
- They also play a major role in the sequestration of ions that are concentrated during cellular dehydration.
- These proteins help to protect the partner protein from degradation and proteinases that function to remove denatured and damaged proteins.
- Dehydrins, also known as a group of late embryogenesis abundant proteins, accumulate in response to both dehydration and low temperature.
- In addition to their synthesis at the desiccating stage of seed, they also accumulate during periods of water deficit in vegetative tissues.
3.3 Signaling and drought stress tolerance
- General responses to stress involve, signaling stress detection .
- Chemical signals e.g. ROS, Ca and plant hormones-induce stress tolerance
- MAPK are important mediators in signal transmission, connecting the perception of external stimuli to cellular response.
- The complexity of signaling events associated with the sensing of stress and the activation of defense and acclimation pathways is believed to involve
- Reactive oxygen species,
- Calcium and calcium regulated proteins,
- Mitogen-activated protein kinase cascades
- Cross-talk between different transcription factors
Induction of drought resistance
- Seed priming
- Use of plant growth regulators
- Use of osmoprotectants
- Silicon application
A number of different seed priming techniques have been developed, such as Hydropriming (soaking in water)
Halopriming (soaking in inorganic salt solutions)
Osmopriming (soaking in solutions of different organic osmotica) Thermopriming (treatment of seeds with low or high temperatures)
Solid medium priming (treatment of seeds with solid matrices) Biopriming